
Chapter 03 - Simultaneity
Download the complete chapter



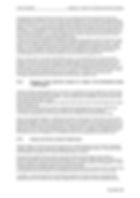

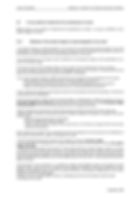

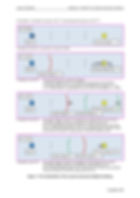
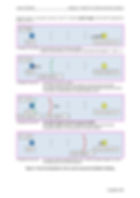
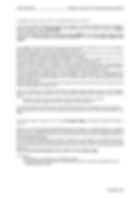
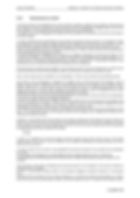
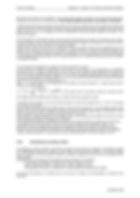
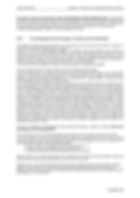
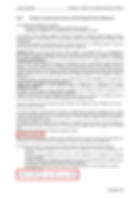
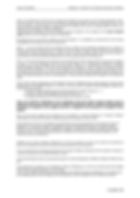

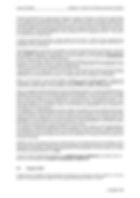
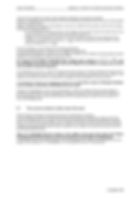

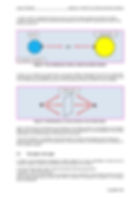
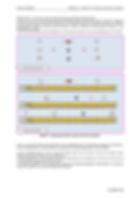
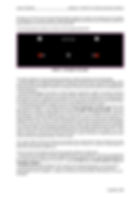
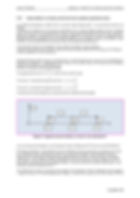
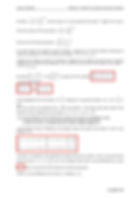
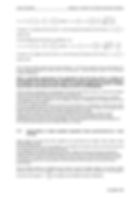

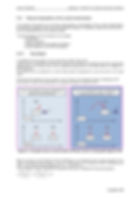
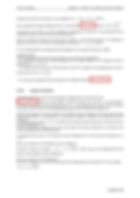
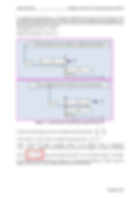

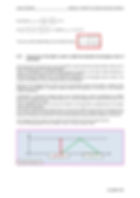
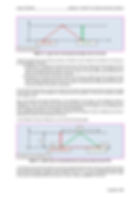
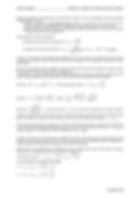
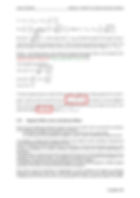
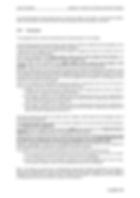

3.1 Introduction
The notion of simultaneity is fundamental, first to better define what the time is and secondly in order to give a fair and proper interpretation of the Lorentz transformation.
3.2 Definition of the simultaneity according to Einstein
Albert Einstein faced the question of the simultaneity of two events with deep and honest reflection.
In “The theory of special and general relativity”, at the beginning of Chapter VIII, Albert Einstein wrote «About the concept of time in Physics»:
«I assume that lightning struck the track of our railway in two points A and B very remote from one another and affirm that these two flashes were "simultaneous". If now I ask you, dear reader, if this statement has a meaning, you will answer me with conviction "Yes". But if I insist and ask you to explain to me in a more accurate way the meaning of this assertion, after some reflection you can admit that the answer to this question is not as simple as it seems at first.
After some time, maybe you will have in mind the following reply: "the meaning of this statement is clear in itself and does not need further clarification; indeed, it would make me think a little while, if I was instructed to prove by means of observations that the two events are simultaneous or not." This answer does not satisfy me for the following reasons. Suppose that a meteorologist has found by perceptive reflections that the lightning must always fall at the same time at points A and B; we would then need to check if this theoretical result matches or does not match the reality. It is the same for all physical assertions where the concept of "simultaneous" plays a role. This concept exists for the physicist only if he has found the possibility to check, in the concrete case, if it is or not accurate. So we need a definition of simultaneity so that it gives us a method allowing us to decide, with the help of experiments, if the two lightning strikes were simultaneous or not. As long as this requirement is not satisfied, I am as a physicist (and as a non-physicist too) the victim of an illusion, if I think I can give a meaning to the affirmation of the simultaneity. (If you do not grant me this, dear reader, with conviction, it is useless to go on.)
After some time of reflection, you could make the following proposal to verify simultaneity. Measure the segment AB right along the track and put in the middle M of this segment an observer equipped with a device (for example two mirrors inclined at 90 °) which allows him to observe simultaneously the two points A and B. If he perceives the flashes at the same time, they are simultaneous.»
In chapter IX "Relativity of simultaneity" of the same book, Einstein wrote:
"So far, our thinking had in view a particular reference body, that we called "the railway". Suppose a very long train moving with a constant speed V.
The travelers on this train will have advantage to use the train as a body of rigid reference (coordinate system), to which they will report all the events. Any event taking place along the railway also takes place at a specific point on the train. The definition of simultaneity can also be formulated in exactly the same way concerning the train as concerning the track. Thus the following question arises quite naturally: are the two events (e.g. the two flashes of lightning A and B), which are simultaneous to the track, also simultaneous with regard to the train ? We will show soon that the answer should be negative.
The travelers on this train will have advantage to use the train as a body of rigid reference (coordinate system), to which they will report all the events. Any event taking place along the railway also takes place at a specific point on the train. The definition of simultaneity can also be formulated in exactly the same way concerning the train as concerning the track. Thus the following question arises quite naturally: are the two events (e.g. the two flashes of lightning A and B), which are simultaneous to the track, also simultaneous with regard to the train ? We will show soon that the answer should be negative.
When we say that the flashes A and B are simultaneous with regard to the railway, we understand that the rays coming from the points A and B meet in the middle M of the distance A - B on the track. But the events A and B are in correspondence with the places A and B in the train. Let M’ be the middle of the segment A - B of the running train. This point M’ do coincide with the point M at the moment when the flashes of lightning occur (seen from the slope), but it moves on the drawing to the right with velocity v. If an observer on the train sitting in M’ was not carried with this speed, he would remain permanently in M and the light rays coming from A and B would reach it simultaneously, that is to say that these two rays would meet at the point where he is located. But in reality, he runs (as seen from the slope) toward the ray of light coming from B, while he flees before the one coming from A. As a result, he will see the ray of light coming from B earlier than the one coming from A. The observers who use the train as a reference body must therefore come to the conclusion that lightning B has occurred previously to the flash A. Thus, we obtain the following important result:
Events that are simultaneous with regard to the railway are not simultaneous with regard to the train and vice versa (relativity of simultaneity). »
I will try to show that this definition of simultaneity (or rather this method to determine if two events are simultaneous) given by Einstein, far from being "so natural" as he said himself further in chapter IX, is wrong and leads to a vision of time which is not good and has put the scientists on a wrong path for more than a century.
3.3 Possible objections to the definition of simultaneity proposed by Einstein
I'll mention a few objections to the definition of simultaneity proposed by Albert Einstein. Some of these objections will be developed in the following chapters.
3.3.1 Assimilation of the event itself and its « optical image »
The first objection, which is probably the strongest, concerns the fact that the observer situated halfway M of AB is not sensitive to the event itself, but to what I call the “optical image” of the event that is to say an electromagnetic wave emitted or reflected by the event.
It can be confusing because there are two types of events:
-
- those sending an optical image (or an electromagnetic wave to be more general) for example a flash, a solar flare, a lit lamp ...
-
those that do not send themselves an electromagnetic signal and require the presence of a source (e.g. the Sun) to be lit and reflect the incident wave. Most of the events are of this type, as for example the impact between two objects.
The examples considered by many authors writing on relativity and Einstein himself (the flashes of lightning) belong to the first category, that is to say that these are events that emit themselves an electromagnetic wave. So it's very easy to make the assimilation, or even the amalgam, between the event itself and its “optical image” which propagates (and can last a long time) and so to confuse the moment when the flash of lightning “flashes” with the moment when an observer receives the optical image.
In the case of the impact between two objects (e.g. two billiard balls), it is easier to distinguish between the event itself (particularly the moment at which it occurs) and the optical image of the event (and the moment when an observer receives the light wave from a source distinct from the objects and reflecting on them in particular at the time of the impact).
Note: it can be already noticed that I'm talking about the moment of an event without mentioning any observer (notion of an absolute time) and of the moment of the receipt of the optical image by an observer (relative concept of reception time mandatorily linked to an observer and that is different for any observers).
3.3.2 Choice of the moment of the middle M’ of A - B in the referential R'
The second objection concerns the choice of the moment of the middle M’ of AB in the referential R'. Einstein writes: “Let M’ the middle of the line A - B of the bandwagon. This point M’ coincides very well with the point M at the moment where the flashes occur, but it moves on the drawing to the right with velocity v.“
Concerning the choice of the moment of the middle M’ of A - B, nothing justifies to take the moment when the flashes occur.
We could consider taking the point M’ coinciding with the point M at the moment when "the rays coming from the points A and B meet in the middle M of the distance A - B on the railway.''
3.3.3 Impossibility of determining the point M’ by the experiment proposed by Einstein - use of absolute time
I now have to develop the previous point that is crucial.
Einstein’s argumentation leading to the affirmation that simultaneity is relative has two essential phases:
-
-the first phase only happens in the referential R (railway) in which the flashes of lightning occur simultaneously and in which lies the point M middle of the segment AB. Both events are reported simultaneous because "rays coming from the points A and B meet in the middle M of the distance A - B on the railway.” This first phase is completely justified and I agree with the proposed method until then. It is important to clarify that we are talking about the point M (spatial position only) and not of the event M because it is assumed that M remains the middle of AB throughout time. The spatial position of M middle of AB space M is sufficient.
-
-the second phase takes place in the referential R' which is linked to the train. Here things become complicated, and I don’t agree with Einstein’s method any longer. In fact, Einstein wrote: "Let M’ be the middle of the line A - B of the bandwagon. This point M’ coincides with the point M at the moment when the flashes occur... ». Thus, M’ is not a point in the space, it is an event, i.e. a spatial position at a given time. It is the equivalent in R' of the point M in R at the moment when the flashes occur. Using Einstein’s method and light signals, it is therefore impossible to determine the point M’. Indeed, there are two ways to determine the event M’ which prove to be both impossible to implement in concrete terms:
-
whether we continuously determine the points A' and B' in R' equivalent of the points A and B in R, in particular at the moment when the flashes occur. The problem is that these two points A' and B' are changing continuously in R' (the train) and it is therefore impossible to deduce instantly the middle M’ especially at the instant when the flashes occur;
-
or we use directly the point M from R to determine the point M’ of R'. However, it must determine the point M’ at a very particular moment which is when the flashes occur. There is still impossibility because at the specific moment when the flashes occur, the point M is not yet informed. It will only be when the two rays from the points A and B reach it. It will be too late because this is not this moment that Einstein chose for his method of determination of simultaneity.
In fact, without realizing it, Einstein uses the absolute time necessary to determine the point M’ at the moment when the flashes occur. Then it's mathematically wrong to come to a conclusion when time is relative by using an absolute time in the demonstration.
Note 1: If one uses the moment when the rays from the points A and B reach the point M to define the point M’ in R' then everything back in order, because the observer in M’ (or rather the event < point M’ at the moment when the two rays arrive in M>) will assert that the two rays from points A and B came up to it at the same time, as stated by the observer M in R.
Note 2: a few authors give a variant of Einstein’s method. They assume that “two flashes occur simultaneously at points A and B of the railway when they coincide with the ends A' and B' of the train”. They take the example of a “passenger sitting in the middle M’ of the bandwagon A’B’”. Everything seems resolved because the middle M’ of the ends A' and B' of the train can be determined in advance. In fact, this is not so simple. Indeed, in order to verify that the length A'B' of the train corresponds to the distance AB on the track (or to put marks at the front and the rear of the train corresponding to the points A and B), the train must be stationary on the railway and located between the two points A and B. Then, when the experiment is carried out and the train is travelling at very high speed, an observer on the railway will consider that the length of the train has shrunk. If we choose as reference A' = A then these will be the points M’ and B' which will be offset from the points M and B, if we choose as the reference B' = B then these will be the points A' and M’ which will be offset from the points A and M and finally, if we choose as reference M' = M then these will be the points A' and B' which will not coincide any more with the events A and B.
Finally if we carry out calculations to determine the position of the points A' and B' on the train in such a way that the apparent distance A'B ' matches the distance AB when the train is in motion, I consider that it is not a “direct” experiment anymore which allows to establish the simultaneity of two events. Indeed, it is then possible to argue that a calculation can determine the position of the point M’ when the signal from B reaches it and then its position when the signal from A reaches it and there is nothing mysterious to find a mismatch between the arrival of the two signals on M’.
Note 3: in fact, what I am trying to demonstrate is that, in an experiment where nothing has been prepared in advance, when two events occur simultaneously on the railway and when a train comes through, the only ACTION which is possible to realize instantly (at the very moment when the two events occur) is to mark the two points A' and B' in the train corresponding to the events A and B. The point M middle of AB is already known but it can’t yet give its information to the train in order to obtain the point M’, because at the time when the two events occur, it is simply not yet aware! (the information teaching that the two events have occurred hasn’t reached it yet).
3.3.4 Presence of two observers instead of a single or the simultaneity known « afterthought »
Instead of putting a single observer M’ on the train, it is sufficient to put two observers A' and B' at the two locations where the flashes will appear. The two observers are equipped with clocks that we take care to synchronize before the experiment. The two observers are instructed to stop their clock at the time where the flash “falls” on them.
They have just to meet to compare their clocks and check "after the fact" that both flashes have rightly fallen simultaneously.
They can also call each other by radio to compare their memorized hours or even to transmit the information to a third party, located in M’ middle of AB, responsible for the comparison.
The only pre requirement for this method is that the two observers have synchronized clocks.
Note: we find the same difficulty to determine the position of the points A' and B' than in note 2 of the preceding paragraph. However, in the present case, it is not necessary to know the middle of A'B'. It is sufficient to install a set of synchronized clocks throughout the train. It will be needed to raise the time given by the nearest clock to the lightning fell in A as well as the time given by the nearest clock to the lightning fell in B. To verify that we have selected the right clock, it is possible to compare the time of this clock with that of its neighbours. The selected clock is the one giving the most anterior time.
3.3.5 Using sound waves instead of light waves
Another objection concerns the choice of light wave (or electromagnetic wave) to define simultaneity.
Imagine a planet on which beings are intelligent but don’t own the sense of vision. On the other hand, these beings are equipped with a highly developed sense of hearing.
Put that at some stage of their evolution, they have made the same reflection that Einstein to determine concretely if two events are simultaneous or not. Naturally, these beings will choose sound waves to determine whether two events are simultaneous using the midpoint of the segment A - B and the same definition as Einstein apart from the choice of the wave.
These beings would therefore declare simultaneous some events which wouldn’t be for a human being with eyes and they would declare non-simultaneous events that would be so for a human being.
Incidentally, I like the example of the two flashes proposed by Einstein because, associated with lightning, there is the thunder that rumbles. Imagine that in the middle of a train are seated side-by-side a "sighted" and a blind person. We consider two flashes hitting the front and the rear of the train. If the two flashes are very slightly shifted temporally in such a way that the sighted person sees them at the same time, the blind person will say that the flash in the front took place before the flash hitting the rear of the train because he will have heard the sound of thunder corresponding to the front flash first. Now suppose the gap of time between the two flashes is such that it is the blind person who simultaneously heard the two noises of thunder at the same time. He will affirm that the two flashes have taken place at the same time. The sighted person will smile and assert that the flash of lightning striking the rear of the train was prior to the flash hitting the front of the train.
The sighted and the blind person won't agree on the simultaneity of events while they are both in the same referential and they have the same atomic clocks.
3.3.6 Are life and death absolute or relative to the observer ?
If instead of two lightning along a track, we take as events:
-
- in point (A) of the railway, at the moment tA,, a black cat is unfortunately crushed near a crossing by a car;
-
- in point (B) of the railway at the moment tB = tA (in the referential of the railway), a white cat is also crushed near a crossing by a truck.
For a fixed observer with regard to the railway, at the middle M of the segment AB, the two events occur simultaneously.
However, for an observer in the train at the point M’ whose Einstein is talking, the two events do not take place simultaneously. The train moving to the point B, the observer in M’ will consider that event B occurs before the event in A.
It is interesting to focus on the precise moment when the observer in M simultaneously receives the signals from A and B because at this time, he will declare that the two cats died while the observer located at the point M’ in the train will assert that only the white cat is already dead (he has already received the signal informing him of its death) but that the black cat is not yet dead (he has not yet received the signal informing him of its death). Also the two observers disagree over the life or death of the black cat.
Is it reasonable to think that two observers can disagree on the life or the actual death of a being? Either the being is actually, physically dead, or it is not yet.
3.3.7 Synchronized clocks in a GR must be synchronized for all GR
Finally the last objection that I make about Einstein’s definition of simultaneity is that it leads to "events that are simultaneous with regard to the railway are not simultaneous with regard to the train and vice versa (relativity of simultaneity)" as wrote Einstein.
Thus, if N clocks (for example two clocks) are fixed in a same GR (Galilean Referential) and have been synchronized, the beating of these clocks will not be considered synchronized by another GR in translation compared to the first, even though we know in advance that the clocks are actually synchronized. In fact, the physical experiment to synchronize or check the synchronization of clocks is performed in advance and then through this check the physicist knows that the clocks will remain synchronized between them.
In Einstein’s vision, the other referentials will assert that the beating of these clocks are not synchronized because he imagines that for each beat, each clock emits a light beam or an electromagnetic signal and that these are the receiving times of the different signals from different clocks which are not simultaneous.
In my vision of simultaneity, a correct definition must comply with the following principle :
"several identical clocks placed in the same Galilean referential and that one took care to synchronize, must be declared synchronized in ALL the Galilean referentials."
This also means that :
“the simultaneity of two events does not depend on the observer. Either they are simultaneous, or they are not.”
3.4 A new method to determine the simultaneity of events
Before giving a new method to determine the simultaneity of events, I will give a definition of the optical image of an event.
3.4.1 Definition of the optical image (or electromagnetic) of an event
I call optical image (or electromagnetic) of an event, the omnidirectional electromagnetic wave that propagates in all spatial directions at the speed of light and which is emitted at the precise moment of the event by a source located at the precise spatial location of the event.
The fundamental error of Einstein, that his definition of simultaneity implies, is the assimilation of an event to its optical image.
An event occurs at a given spatial position and at a given time and afterwards, there is nothing.
The optical image is a wave that evolves, propagates away from the event in all directions and that lasts over time (sometimes very long as for the stars, for example).
In the vision of special relativity, different observers will not agree on the simultaneity of two events:
-
A first observer will say that the event E1 took place before the event E2
-
A second observer will say that the event E1 was held simultaneously with the event E2
-
Finally, a third observer will say that the event E1 took place after the event E2.
In fact, these three observers are sincere and are not wrong about what they have seen, but they are wrong about their interpretation.
This is not the event E1 itself, which took place before, simultaneously or after the event E2, but it is the optical image of the event E1 that took place before, at the same time or after the optical image of the event E2 which is not the same thing at all and it changes everything.
Take the example of a star that is a billion light-years from Earth and died 500 million years ago.
Imagine several observers distributed along the distance between the position occupied by this star and the Earth:
-
- Some will argue that the star is long dead;
-
- Some will argue that the star is dying;
-
- Finally, other observers closer to the Earth (and on the Earth) will argue that the star does exist since they see it!
Which observers are right? This is important because the stakes are not only about the simultaneity of two events, but about life or death, the existence of a star.
I like this example because the answer to the question is obvious: the star is dead.
In fact, here again the observers do not give information about the star itself, but about the optical image of the star.
I can say with determination that the star is really dead because its optical image is, after all, as data on the star and not the star itself. Moreover, it is possible to imagine that a space probe was sent by the Earth long before the death of the star, filmed all the death of the star, and then returned to Earth where scientists have watched the film while determining, by "earth time" in which period occurred the death of the star.
The next point is very important: it is possible to obtain three different visions of the state of a star (already dead, dying and not yet dead) by three different observers using light signals and three different chronological orders of the two events (anteriority, simultaneity, posteriority) by the same observers who still use light signals.
However, the real, physical state of a star is unambiguous and the chronology of events is also unambiguous as unique.
3.4.2 Story from Stephen Hawking
To support the above remarks, I will discuss a story that Stephen Hawking has written in his book A briefer history of time:
“Most of these authors (science fiction writers) don’t seem to have realized the fact that if you can travel faster than light, the theory of relativity implies you can also travel back in time (…). The key to this connection is that the theory of relativity says not only that there is no unique measure of time on which all observers will agree but that, under certain circumstances, observers need not even agree on the order of events. In particular, if two events, A and B, are so far away in space that a rocket must travel faster than the speed of light to get from event A to event B, then two observers moving at different speeds can disagree on whether event A occurred before B, or event B occurred before event A. Suppose, for instance, that event A is the finish of the final hundred-meter race of the Olympic Games in 2012 and event B is the opening of the 100,004th meeting of the Congress of Proxima Centauri. Suppose that to an observer on Earth, event A happened first, and then event B. Let’s say that B happened a year later, in 2013 by Earth’s time (1). Since the Earth and Proxima Centauri are some four light-years apart, these two events satisfy the above criterion : though A happens before B, to get from A to B you would have to travel faster than light. Then, to an observer on Proxima Centauri moving away from the Earth at nearly the speed of light, it would appear that the order of the events is reversed : it appears that event B occurred before event A (2).
This observer would say it is possible, if you could move faster than light, to get from event B to event A (3). In fact, if you went really fast, you could also get back from A to Proxima Centauri before the race (4) and place a bet on it in the sure knowledge of who would win!”
Stephen Hawking’s text being not quite clear to me, I will distinguish two versions depending on the interpretation of the sentence (1):
-
- 1st version : event A occurs in 2012 TY (Terrestrial Year) and occurs in 2013 TY (it is probably the case mentioned by Stephen Hawking)
-
- 2nd version : event A occurs in 2012 TY (Terrestrial Year) and reaches the Earth in 2013 TY.
1st Version: event A occurs in 2012 TY and event B occurs in 2013 TY.
In this case, Stephen Hawking says that for an observer on Proxima Centauri leaving the Earth at nearly the speed of light, it would appear to him that the order of the events is reversed: it appears that the event B occurs before the event A.
I agree with Stephen Hawking if we insist on the word "appears" and we say that the order given by the observer is not the order of the events themselves, but that of the optical image of the events.
Then Stephen Hawking wrote that "this observer would say that it is possible, if you could move faster than the speed of light, to move from the event B to the event A."
He further added that "if you've really moved very quickly, you might also be back on Proxima Centauri before the race and bet with the certain knowledge of the winner!”
Here I don’t agree with Stephen Hawking any longer. It is impossible for an observer, even if he is in a spaceship going much faster than light, to move from the event B to the event A.
However, for this observer, it is possible to reach the optical image of the event A and therefore to know the winner of the race and then return to Proxima Centauri to bet BEFORE the optical image of the event A (the event A is the end of the race on Earth) have reached Proxima Centauri.
Here we are speaking of transmission, of the "propagation" of the information and being informed of an event before the information about this event reaches a certain point. But it is impossible for an observer starting from Proxima Centauri in 2013 to arrive on Earth in 2012 even traveling much faster than the speed of light.
Besides, the traveler into his spaceship can change nothing to the events which occur during the final of the hundred meters on Earth in 2012.
Note: in the sentence "you might also be back on Proxima Centauri before the race," the term "before the race" is very vague (at least for me). When Stephen Hawking wrote "before the race”, does he mean :
-
before the event A has occured, that is to say, the finish of the race on Earth ?
-
the optical image of the event A reaches Proxima Centauri ?
It seems that Stephen Hawking thinks of the second option which is, incidentally, the only possibility.
But this shows that there is confusion between the event itself and what I call the optical image of the event.
2nd version: event A occurs in 2012 TY and the optical image of the event B reaches the Earth in 2013 TY.
Although I do not think Stephen Hawking described this version, it is interesting because, unlike the previous case, the spacecraft leaves from the event B and actually arrives physically to the event A that is to say, on Earth at the time of the arrival of the race. This of course is possible because the spacecraft is faster than light.
So in this version, everything said by Stephen Hawking is true (the traveler in his spaceship can leave from the event B and reach the event A, return to Proxima Centauri and be sure to win his bet) except that the very beginning of his story asserts that the event A occurs in 2010 TY and the event B occurs in 2013 TY.
This is not the event B itself that occurs in 2013 TY, but its optical image.
Conclusions:
-
simultaneity is not a relative but an absolute concept;
-
the absolute nature of simultaneity implies that every travel in the past is impossible even by traveling faster than light.
3.4.3 Simultaneity by contact
The first principle of simultaneity that I give is that the observer located at the position of the event at the moment it occurs is evidence (is the reference) and his observation findings prevail over all the other observers. This observer has "authority" over all the other observers.
To speak in a common language this means that "the witness who was there at the time of the facts is the most reliable.”
In order to determine the simultaneity of two events, according to this principle, it is necessary to take two observers, the first observer being located at the position of the first event at the instant it occurs and the second observer being located at the position of the second event at the moment it occurs.
Finally, both observers must be in the same inertial frame in order to have clocks with the same period and consequently remaining synchronized.
This last requirement is feasible if we consider any Galilean referential so that all the events in the universe are "spotted" in this referential, that is to say that one can assign them a spatial position (x, y, z) and a time t. Considering that this referential is "fulled” of observers in 3 dimensions (linked to this referential), the two events will effectively correspond to two observers.
Once the above is placed and accepted, it only remains to each of the two observers to note the time at which the event occurs at the position where he is and then compare the results by radio.
Note: all the reasoning is considered "out of gravitation", that is to say without any gravitational field.
Let’s return to the “experiment” proposed by Einstein with a very long train (for example, long of several “light-minutes” measured at rest in the reference frame of the railway) and a railway long of several “light hours”. Let the points A and B of the railway be known in advance separated by a "light minute" but we do not know when lightning will strike the points A and B. Suppose that we have passengers spread in the train cars and being observers on the train.
At the very moment when the two lightnings strike point A and point B, there are also two passengers A 'and B' who watch directly the flashes of lightning.
At this very moment, the point M middle of AB on the railway has not yet received the information that the two lightnings fell. Similarly, the point M' middle of A’B’ is not yet known because the two passengers A' and B' who observe the two flashes have just been known.
If we have synchronized all the clocks in the railway and the train at the time of the departure of the train, it is no longer the case, at the moment when the two flashes occur, between the clocks of the railway and those of the train.
However, if we accept that all the clocks of the railway remained synchronized with each other and that all the clocks of the train remained synchronized with each other, then the two observers A and B of the railway, as well as the two observers A' and B' of the train would realize they have memorized the same time when they compare their results:
-
tA = tB
-
tA’ = tB’
In short, it is essential to note the position and the moment when each event occurs in the same inertial frame. This is what I call the method of the observer standing at the same place and at the same time as the event.
In order to make more concrete, more palpable the previous principle, we can define the simultaneity by contact.
The principle of simultaneity by contact states that two bodies beginning to be in contact are simultaneously at the same place (at the contact time, at least concerning the atoms involved in the contact).
This principle is particularly interesting and bring some light on the "paradox" of the twins invented by thephysicist Paul Langevin.
For this, I will use Paul Davies’s article in the excellent magazine "Scientific American" of November 2010:
"Suppose Sam and Sally are twins. Sally embarks on a rocket and travels at high speed to a nearby star, turns around and comes back to Earth, while Sam stays at home. For Sally, the trip lasted a year. But when she returns, her brother is nine years older. Sally and Sam do not have the same age, although they were born the same day: in fact, Sally jumped nine years in the future of the Earth.”
I totally agree that Sally has aged more slowly than her twin brother and is only twenty-one years old when she returns to Earth while her brother is thirty assuming that the journey began when they were twenty years old, but I am against the final interpretation that says Sally jumped nine years in the future of the Earth.
My interpretation is that Sally's body, like all physical phenomena occurring in the rocket, had a slower rythm (an atomic clock will have its period actually, physically bigger and therefore its frequency lower) but Sam and Sally were continually in the same present.
Rather than a jump in the future, it is rather as if Sally had taken drugs which biologically slow the aging of her body while living, like her brother, the same events (it is just the duration between the events which is different for Sam and Sally). The difference is that the slowing of the clocks depending on their speed is a physical effect which therefore applies to ALL physical phenomena and not merely a biological process.
The principle of simultaneity by contact will help me support my point.
Let’s go back to Einstein's experiment of the train on its railroad. This experiment is interesting because the train, instead of going away from the track as the rocket is going away from the Earth, is still on the track. Suppose that the railway is very long, for example several light years. Suppose that the train has reached a constant speed of V = 0,9.c and that we synchronize the clocks of the train with those of the railway at a certain time t0 = 0.
After Dt = 10 years spent in the referential of the railway, the train traveled D = 9 light years and the clocks in the train indicate :
4.36 years, that is to say that a person in the train would have aged only 4.36 years while a person on Earth would have aged ten years.
According to Paul Davies, we can say that the person on the train jumped from 10 - 4.36 = 5.64 years in the future of the Earth.
Now suppose that after these ten Earth years, the train kept its speed 0,9.c and a flexible plastic plate sticks out of a train window. Assume that along the track, a flexible plastic plate is maintained so that the two plates touch each over after ten years of travel of the train.
What does the contact between the two plates mean, one traveling at the speed of the train and the other remaining immobile wih regard to the railway?
If we accept the principle of simultaneity by contact, this means that the two plates which collide are simultaneously at the same place. They are at the same present time during the contact. The experiment of the two plates being able to be repeated throughout the track, this means that the clocks of the train and those of the track are continuously at the same present time but with different rhythms, different periods. This point will be much more developed in the next chapter on time.
3.4.4 Simultaneity by sliding contact
Simultaneity by sliding contact is only there to support the fact that two "objects" in permanent contact but nevertheless moving with respect to each other, are continuously present in the same present even if the atoms of the first object vibrate at a different frequency from the one of the atoms of the second object.
As examples of movements with permanent sliding contact we can mention:
-
- A bobsleigh, ice skates in contact and in motion relative to the ice;
-
- Skis, a snowboard, sled in contact and in motion relative to snow;
-
- The catenary of a train in contact and in motion relative to the electrified cables.
If we take the example of a "sliding" train on the rails of a railway, the interpretation I propose is the following :
The clocks of the train and those of the track effectively indicate different times. Nevertheless this effect combined to the fact that the real, physical period of the clocks on the train is different from the one of the clocks along the railway, as they are altered by their speed relative to the Preferred Frame of Reference, results that the train and the railway are permanently in the same present.
All that will be developed in detail in the next chapter on the time.
3.4.5 The messenger and the message - the wave and the information
This method of determining simultaneity is very interesting and very instructive because it is based on the method proposed by Einstein but it improves it.
Indeed, we will use electromagnetic signals, as Einstein used light signals, but enriched by information. We can already notice that Einstein does not use any device at the positions A and B where the lightning occur because they emit, by their nature, a bright light that will be seen by the middle M of AB. However, if the events were objects in unstable equilibrium falling to the ground, a device that emits a light signal toward the point M would have to be added.
It is this device that I propose to improve in order to achieve an absolute simultaneity.
First of all, allow me to tell a story, hoping not to try the patience of the reader.
The story takes place in a kingdom surrounded by three neighboring countries: one in the North, another in the East and the last in the South. The wise king built his main castle in the center of his kingdom to respond as quickly as possible and in a balanced way in case of attack. He also built three forts at strategic locations along the three borders.
The three countries surrounding his kingdom joined forces and decided to attack at close dates. The king's messengers are expected to leave the fortresses and go and inform the king in case of attack. This is what happened, and the day after the attack, a first messenger arrived in the morning from the North, a second messenger arrived in the afternoon from the East and finally, the last messenger arrived in the evening from the South. By late evening, the king made the point and relied on arrival times of the messengers to deduce when the three fortresses were attacked. But his counsellor, wisely advised him to listen to what the messengers had to say although they were exhausted. The first messenger informed that the assault of the Northern fortress began the night before but he had ridden all night without stopping and he arrived the day after the attack in the morning. The second messenger informed the king that the fortress of the East was attacked the day before in the early afternoon. Finally the third and last messenger informed the king that the fortress of the South was attacked the day before in the morning, but he had great difficulty in reaching up to the castle of the king and has therefore arrived in the evening of the day after the attack. The king thanked the messengers, congratulated his counsellor and decided to change his strategy and launch troops to the fortress of the South in priority.
This story is intended to emphasize that when receiving information, whatever its nature, there is the messenger and the message itself.
For a light beam, there is the electromagnetic wave itself and the information carried by the wave.
Thus, it is possible to improve Einstein’s method putting an identical device at the points A and B including an atomic clock, a source capable of emitting an electromagnetic wave and an apparatus for using the wave as carrier of the following information:
-
- A unique number of the tag that sends the signal (tag No. B ...);
-
- A unique number that identifies the event (event No. E ...);
-
- The date and exact time of the event given by the atomic clock.
With this device, any observer belonging to any referential can decode the electromagnetic signal and properly know well the chronological order of the arrival of the events.
Important note : even if the time between the two events, obtained through the information of the two signals, does not match with what the clocks linked with his referential would have provided, any observer owns the real and unique chronological order between the events and the information of their simultaneity or non-simultaneity.
3.4.6 Armada of synchronized clocks in the Privileged Frame of Reference
The purpose of this method is to acquire:
-
- A unique chronological order of all the events of the universe;
-
- In particular to determine in an absolute way the simultaneity of events.
The principle of this method consists in having an "armada" of clocks regularly spaced in three-dimensions (sort of cubic lattice with a clock at each vertex) and all belonging to the Preferred Frame of Reference.
The first step consists in synchronizing all the clocks.In doing this, it is sufficient to take a clock as a reference and then to synchronize the other clocks with regard to it.
Important note: we will see at the end of this chapter on simultaneity that it is impossible to synchronize two spatially separated clocks and in the same inertial frame. Any observer belonging to this Galilean referential thinks that the clocks are synchronized but in reality they are not. It is an apparent synchronization.
The Preferred Frame of Reference is the only referential in which it is possible to really synchronize clocks. To be more specific, it is possible to synchronize the clocks in the Preferred Frame of Reference and then submit them to an acceleration and deceleration so that they all have the same constant speed relative to the Preferred Frame of Reference while remaining synchronized with each other (but they will be not synchronized with the clocks remained in the Preferred Frame of Reference).
It is also possible to synchronize clocks in any inertial frame having knowledge of the speed of the frame relative to the Preferred Frame of Reference.
Indeed, the knowledge of the Preferred Frame of Reference is mandatory to actually synchronize clocks.
As modern science does not have sufficient knowledge of the Preferred Frame of Reference, it is impossible to synchronize two clocks spatially separated.
However, if the Preferred Frame of Reference exists (as this theory maintains), it is possible to have in mind the concept of synchronized clocks belonging to this reference frame.
I'll give two methods to synchronize two clocks located in the Preferred Frame of Reference but there are a lot of other methods.
1) The first method to synchronize two clocks is to determine the middle M between the two clocks located at the points A and B. The two clocks have an electromagnetic waves transmitter and a signal is sent by the transmitter of each clock when each of the two clocks marks a certain time agreed in advance (for example 15 hours).
The observer at point M notes the time difference Dt between the arrival times of the two signals by applying a positive sign if the signal from B arrived first and a negative sign if the signal from A arrived first.
We have just to set the clock B using the following formula:
.
Note : the clock owned by the observer at the middle M does not need to be synchronized neither with the clock in A, nor with the clock in B, because the method only requires a time difference.
2) The second method to synchronize two clocks consists in applying the following procedure:
-
- At time tA_transmission measured by the clock in A an observer in A emits an EM signal to the clock B;
-
- At time tB_reception measured by the clock in B the observer in B receives the previously transmitted signal;
-
- A person measures the distance dAB between the points A and B that remains constant as the two clocks still belong to the Preferred Frame of Reference;
-
- The observer in B synchronizes his clock, by taking the clock in A as a reference, using the following formula :
.
Note: This method can work with any signal sent between the points A and B (electromagnetic wave, acoustic wave). There may even be an object covering the distance AB as, for example, a train. The only constraint is that the signal or object travels the distance AB with a constant speed module with respect to the Preferred Frame of Reference.
This remark also applies to the first method using two signals or two objects of the same constant speed relative to the Preferred Frame of Reference.
By applying one or the other method of synchronization, it is possible to synchronize all the clocks belonging to the Preferred Frame of Reference.
Note 1 : we can remark that this method may be faster than expected because the number of synchronized clocks may grow into 2N where N is the number of steps. Indeed, the first clock taken as a reference allows synchronizing a second clock. The two synchronized clocks can be used to synchronize two others thereby obtaining four synchronized clocks, then eight, etc.
Note 2 : the synchronization of all clocks can be performed "at the speed of light" if the second method is used. It is just necessary that the first clock taken as a reference transmits a powerful electromagnetic signal at a date and time scheduled in advance. If care is taken to number all clocks with the number of steps in x, y and z with respect to the reference clock (or directly the distance from the reference clock) then once every clock receives the powerful electromagnetic signal (wherein can also be encoded date and time of transmission), it may be synchronized with respect to the reference clock.
Once all the clocks belonging to the Preferred Frame of Reference are synchronized, for each event taking place at the spatial position of a clock, this clock emits a powerful electromagnetic signal with the following information :
-
- A unique number identifying the clock that sends the signal (clock No. H ...);
-
- A unique number that identifies the event (event No. E ...);
-
- The date and exact time of the event given by the atomic clock.
Thus, any observer, belonging to any referential, who will receive several signals sent by different clocks, will be able to find the unique chronological order of events by decoding the information contained in the signals and not in relying on the moments of reception of the signals.
Now, we can also imagine the existence of N armadas of clocks belonging to N distinct Galilean referentials, the clocks of a given armada being synchronized with each other.
For doing this, there are N armadas of synchronized clocks all belonging to the Preferred Frame of Reference. Then, each armada is accelerated and then decelerated to reach the desired speed relative to the Preferred Frame of Reference. Indeed, we obtain an armada of clocks synchronized with each other, but whose identical period is different from the one of the clocks remained in the Preferred Frame of Reference.
Whatever the chosen Galilean referential to host the armada of clocks, the clocks will provide the same chronological order and the same events reported as simultaneous.
The only data that will change according to the chosen Galilean referential is the number of cycles (or number of periods) of the clocks between events.
The following figure shows what several groups of clocks belonging to different Galilean frames would give.
This requires the existence of a Preferred Frame of Reference in which the clocks have their fastest beats (the real, physical period is the shortest).
In any other Galilean referential moving relative to the Preferred Frame of Reference, the clocks have all the beats slower than the speed of the referential is high compared to the Preferred Frame of Reference (the period is physically larger) till the limit of the speed of light c for which there are no beats (infinite period).
The chronological order of events is preserved regardless of the Galilean referential and two simultaneous events are simultaneous for all Galilean frames.
3.5 Experiment or paradox EPR – instantaneity – simultaneity
I can’t avoid mentioning what is commonly called the EPR paradox, named after its authors: Einstein, Podolsky, Rosen.
Einstein and his colleagues propose a thought experiment in an article in 1935.
This experiment suggests considering two systems I and II that interact between t = 0 and t = T, then it is assumed that there is no longer any interaction between the two systems.
It is also assumed that the state of the two systems was known before time t = 0. It is then possible to calculate, thanks to the Schrödinger equation, the state of the combined system I + II at any moment, in particular for t > T. However, it is impossible to calculate the state of one of the two systems after the interaction. Einstein and his colleagues then assume that a physical quantity A belonging to system I is measured. Through Y wavefunction of the combined system I + II, the state of the second system can also be determined. The authors then noted that, since at the moment of the measurement of the system I the two systems do not interact any longer, no real change can happen to the second system regardless of what is done to the first system.
Such is, briefly summarized, the EPR paradox, which involves a concept dear to Einstein : the "principle of locality" also known as the principle of separability which states that distant objects can not directly influence one another. An object can only be influenced by its immediate environment, principle coming from the special relativity.
This principle proves to be challenged by quantum physics, in particular the quantum entanglement phenomena.
Quantum entanglement is a phenomenon observed in quantum mechanics in which the quantum state of two objects is to be described globally, unable to separate an object from another, although they may be spatially separated. When two systems - or more - are placed in an entangled state, there are correlations between the observed physical properties of the two systems that would not be present if one could assign individual properties for each of the two objects S1 and S2. Therefore, even if they are separated by large spatial distances, the two systems are not independent and {S1 + S2} should be considered as a single system.
A famous experiment conducted by Alain Aspect and his team in 1981-82 was to generate two entangled photons. Measuring the polarization of one of the "twin" photons instantly determines the polarization of the other photon.
My thought focuses on the notion of immediacy. Since the measurement of the polarization of the first photon instantly determines the polarization of the second photon, it means that in a given referential (the laboratory), polarization measurements of the two photons can be performed simultaneously (the two notions are very close, almost synonyms).
But then, using Einstein’s method for determining the simultaneity of two events, one may also be in a spacecraft in the middle of the two devices performing the measurement of the polarization of the photons at the moment when they perform the measurement.
If the spacecraft is moving towards the first photon, then the pilot of the ship will declare that the measurement of the polarization of the first photon took place before the measurement of the polarization of the second photon and then it no longer seems to be a paradox to this observer.
What I just would like to say is that Einstein’s assertion, as part of special relativity, claiming that simultaneity is relative also amounts asserting that immediacy is also relative and therefore the instantaneous determination of the polarization of second photon is relative to the observer.
Note: we consider the same experiment but with the following variation. The device generating the two entangled photons and the two devices measuring the polarization of the two photons are in a single referential (the laboratory), but the measurement of the polarization of the second photon is performed with a small time offset from the measurement of the first photon in the laboratory frame. To an observer belonging to the laboratory frame, the measurement of the polarization of the first photon took place before that of the second photon, and this was that measurement which influenced the polarization of the second photon.
To an observer in a spacecraft starting from the middle of the position of the two photons at the time of the measurement of the polarization of the first photon (or second photon) and heading at high speed (nearly the speed of light) toward the device measuring the polarization of the second photon, the measurement of the polarization of the second photon will take place before the measurement of the polarization of the first photon and he will conclude that it is the measurement of the second photon that caused the determination of the polarization of the first photon.
The choice of the referencial appears to determine which of the two photons, by the measurement of its polarization, caused the determination of the polarization of the other photon. Causality between the measurement of the polarization of a photon and the determination of the polarization of the other photon appears inverted according to the choice of the referential. This seems to call into question the principle of causality.
Additional note: if the observer knows or assumes that it is the measurement of the polarization of the first photon that caused the determination of the polarization of the second photon, he will infer that an observation at the present moment (the measurement of the polarization of the first photon) will impact the state of the second photon in the past.
As part of a theory asserting the existence of a Preferred Frame of Reference the problem does not arise any more because the concept of simultaneity is ABSOLUTE.
3.6 Doppler effect
Looking back on Einstein’s initial experiment, the observer on the track and the observer on the train appear to be equivalent with regard to the two lightning hitting the tracks in A and B.
In fact, the two observers, alone in their Galilean referential, are actually equivalent.
However, compared to the two events which are the lightning hitting the track, they are not equivalent due to the Doppler effect.
Indeed, suppose that instead of two flashes, we put two LASERS at the points A and B of the railway, emitting at a single frequency n0 :
-
- For the observer lying on the track in the middle of the points A and B, the light that he will receive from the two LASERS will be at the same frequency n0;
-
- However, for the observer in the train moving from point A to point B, the photons from the LASER in A will have the frequency n0 - nDoppler, while the photons from the LASER in B will have the frequency n0 + nDoppler.
From this reflection, we can deduce the following statement:
Let two events take place in A and B in any Galilean referential R0.
We assume that these two events are an emission of light by two identical monochromatic sources located in the referential R0 (that is to say still in R0).
So, among all the Galilean referentials with a speed vector colinear to ( ), only one referential will have an observer who will measure the photons arriving from the two sources with an identical frequency.
This referential is the one in which the events took place (that is to say the referential where the two sources are stationary) and ONLY an observer of this referential (in particular the one located in the middle of the points A and B) is "authorized" to establish the simultaneity of the two events.
This statement is already very important in itself because it provides a mean to distinguish between several Galilean referentials COMPARED with two events.
However, this statement is much more fundamental. It allows to foresee that the Doppler effect is actually the visible effect of the composition of speeds for the light. This is what the two chapters on the "Equivalence of the Lorentz transformation and the Doppler effect for light" will try to show in a much more detailed way.
​
​
3.7 Two sources located on either side of the track
Albert Einstein proposed an experiment where two lightnings hit a railroad.
It is also possible to perform an experiment where two sources are positioned on either side of the track. In the referential of the railway, the two sources always send a flash simultaneously. Suppose that both sources are sending a flash when an observer on the train is at the level of the two sources. Likewise, let’s assume that a small receiver is placed in the middle of the track at the level of the two sources (see figures below).
Then it is undeniable that the receiver in the middle of the track will receive two flashes simultaneously and the observer on the train will also see two flashes simultaneously.
This example shows that the simultaneity or non-simultaneity is not linked to the referential but to the position of the observer AT THE MOMENT OF THE RECEPTION OF THE SIGNALS.
Thus, in the method proposed by Einstein to establish the simultaneity of two events, according to the spatial position of the two sources, the two events will be reported to be simultaneous by the observer of the railroad and not by the observer of the train (Einstein’s case) or simultaneous by both observers (case presented above).
Now if the simultaneity of two events was actually relative to the observer's frame of reference, all the events simultaneous in the referential R (railway) should not be simultaneous in the referential R' (train).
I think that this sole argument could infer that simultaneity is not a concept relative to the referential of the observer and is therefore absolute.
​
3.8 Einstein’s concept of simultaneity depends on the relative position of the transmitters at the time of emission and of receivers at the time of reception
In fact, Einstein’s concept of simultaneity depends on the relative positions of the transmitters (sources of events) and receivers.
Books or scientific popularization magazines often give the following example:
- A first event E1 occurs on Earth the December 12, 2012 at 12 o'clock GMT;
- A second event E2 occurs on Alpha Centauri on the same date;
- An observer residing on Earth will say that event E1 took place before the event E2 because it will take about four years for the "optical image" of the event E2 arrives to him;
- An observer residing in the vicinity of Alpha Centauri will assert that the event E2 took place before the event E1 because it will take about four years for the "optical image" of the event E1 arrives to him;
- Finally, a pilot in a spaceship carrying a hover in an area midway between the Earth and Alpha Centauri will claim that the two events are simultaneous, as it will take two light-years for each of the events to reach him.
In fact, if we consider two lamps fixed in the same Galilean referential R and the two lights flash simultaneously in the referential R, then the flashes will be seen simultaneously by all receivers lying in the median plane (equidistant points) of the two lamps at the time of receipt of the two flashes.
Again, the fact that the simultaneity only depends on the relative position of transmitters and receivers shows that the reasoning which consists to declare the simultaneity or non-simultaneity of two events depending on the relative speed of the observer is erroneous.
To be very synthetic, it is possible to say that Einstein’s concept of simultaneity and the method for determining the simultaneity of two events are in fact just a theory of the transmission and reception of electromagnetic signals.
3.9 Two lights in the night
A variant of the experiment proposed by Albert Einstein is to use a transmitter (a lamp) and two receivers instead of using two transmitters (two lightnings) and a receiver.
In his book “Was Einstein right?” Clifford Will offers the following experiment:
"Top figure : View from the ground.
Master light source midway between two observers emits a light flash. Each observer sets his clock to read the same agreed-upon amount when flash is received. They agree the reception of the flashes was “simultaneous”.
Bottom figure : View from a train moving past at one-third the speed of light.
Clocks on the train have previously been synchronized using a similar technique on the train. Speed of the light flash as seen by the train observers is the same as seen by the ground observers. Leftward moving signal therefore reaches ground observer "before" rightward moving signal, because it has less distance to travel.
Thus, two events seen to be simultaneous on the ground are seen to be not simultaneous on the train.
First, we must admit that this experiment is very destabilizing for an advocate of absolute simultaneity, since unquestionably the right receiver is reached by the light signal after the left receiver.
A very important point to note is that the figure "seen from the train" and all the Clifford Will’s reasoning are solely based on two assertions:
- The first assertion is: "the speed of the flash of light seen by the observers in the train is the same as the flash seen by observers on the ground";
- The second assertion, more subtle, less conspicuous because apparently "self-evident" is to assume that all the clocks in the train are really synchronized.
Suppose now that the source emits electromagnetic signals not visible to the naked eye (for example at a frequency of 100 MHz) instead of visible light signals and that when the two receivers receive the signal emitted by the first source, they emit a light flash.
The slight modification of the experiment proposed by Clifford Will offers several advantages:
- The first benefit is to reassure the defender of absolute simultaneity that I am because Clifford Will’s experiment seems less destabilizing seen from this angle. With two lamps lighting up simultaneously in the referential of the railway, it seems more difficult to declare these two events non-simultaneous in another framework;
- The second advantage is to fall back on Albert Einstein’s experiment. Indeed, if we want to finish the reasoning of the modified experiment, we must manage to determine whether the two flashes emitted by the lamps of the two receivers are simultaneous or not. They are simultaneous in the referential of the railway. We have to determine if they are so in the referential of the train. So we fall back exactly on the experiment proposed by Einstein which we have already expounded at length;
- The third advantage of this modified experiment is that the "mechanism" of ignition of the lamp of the two receivers is "hidden", is not visible. We only see "two lamps light up in the night." Thus, the discourse on the light and constant speed can be left out. Initially, it will perhaps provoke a smile with the supporters of relative simultaneity who will think that it is not because we do not see the electromagnetic signal that triggers the lighting of lamps that it cannot be used to hold reasoning. To reveal the back of my mind, I will offer the experiment of putting two synchronized clocks spatially separated on the railway. When the two clocks mark midnight, a lamp positioned at each clock lights up. In this experiment, we will also get "two lamps light up in the night" simultaneously in the referential of the railway. What is the fundamental difference with the experiment offered by Clifford Will? In both cases, we get two lamps that illuminate simultaneously in the referential of the railway, but in the second experiment, no reasoning on the light is to hold. A simple mechanism for getting synchronized clocks provides simultaneous ignition of the two lamps.
Two lamps placed along the railway and provided with synchronized clocks simulate the same experiment as the one proposed by Clifford Will. Both lamps not light up on receipt of a signal light, but when their clock shows a certain time.
Now we come to the essential points of the experiment offered by Clifford Will:
- The first point is to assume the same speed flash seen by the observers in the train and observers on the ground. We will see in the two chapters on the equivalence of the Lorentz transformation and the Doppler effect for light that it is not true. In reality, the light has a constant apparent speed (or seemingly constant);
- The second point is really essential in the context of the proposed experiment. It is to say that the clocks are synchronized in the train. In fact, an observer in the train believes that his clocks are synchronized, but he is mistaken. In reality, the clocks are not synchronized. We'll see why in the next paragraph.
3.10 Impossibility to actually synchronize two spatially separated clocks
The method advocated by Clifford Will in his book “Was Einstein right ?” to synchronize clocks is as follows:
"Consider two observers on the ground, equidistant from a central master observer and on opposite sides. The two wish to synchronize their clocks, so they agree beforehand that when each receives a light flash emitted in all directions from the master observer, he will set his clock to a prescribed time. Because they are equidistant from the master observer and the speed of light is the same in both directions, they naturally assume that they receive the signals simultaneously.”
The proposed protocol is acceptable in the context of Einstein’s special relativity.
However, this protocol will not lead to obtain two synchronized clocks within the theory of a Preferred Frame of Reference. We will see why.
Suppose that the central clock H'0, the left clock H'1 and the right clock H'2 are all in the referential R’ and that the distance L' separating the left clock to the central clock is the same as the distance between the central clock of the right clock.
At time t’0, the clock H'0 transmits its signal.
The signal sets the time to reach the two other clocks.
The clock H’1 receives the signal at the time : .
The clock H’2 receives the signal at the time : .
The left and the right observers conclude that their clocks are synchronized.
Let us now see what happens in the Preferred Frame of Reference PFR which is the REFERENCE.
Preliminary Reminder: in the Preferred Frame of Reference theory (the theory of creatons and vortex is a “Preferred Frame of Reference” theory), the rules moving relative to the Preferred Frame of Reference PFR have really, physically their length shorter by a factor g than the rules (of the same construction) in the Preferred Frame of Reference PFR and the clocks in motion relative to the PFR have really, physically their period bigger by factor g than the clocks (of the same construction) in the Preferred Frame of Reference PFR.
The referential R’ being in movement with regard to the Preferred Frame of Reference at the speed V = VR’/RP, the rules in R’ have physically their length L’ shorter than LPFR the one of the rules in PFR by the factor and the clocks in R’ have physically their period T’ bigger than TPFR the one of the clocks in PFR by the factor .
We have thus the following relations : .
For a given length, the number of rules M’ of length L’ measured in R’ and the number of rules MPFR of length LPFR measured in PFR are linked by the relation : .
Likewise, the number of cylces N’ of period T’ measured in R’ (what we call the time t’) and the number NPFR of periods TPFR measured in PFR (what we call the time t) are linked by the relation : .
By calling and we deduce from the relations
the following relations :
or .
Having established this, the duration measured in R’ gives the duration in PFR.
Now, let’s come to the essential point : within the duration Dt, the three clocks have moved in the Preferred Frame of Reference by the distance .
Thus, as the Preferred Frame of Reference is linked to the medium of propagation of light :
-
to reach the clock H’1, the light must have covered a distance smaller by d;
-
to reach the clock H’2, the light must have covered a distance bigger by d;
In the Preferred Frame of Reference, the reception times of the light by the clocks H’1 and H’2 are (taking t0 = t’0) :
Conclusion : an observer in the referential R’ believes that the two clocks H’1 and H’2 are synchronized (since we have ) but in the Preferred Frame which is the REFERENCE we have that is to say that the two clocks are not really synchronized.
Remark : the time difference for the clock H’1 is (taking t’0 = t0) :
The gap t’1-t1 is positive thus the clock H’1, which has been set thanks to the formula , in reality is fast.
The time difference for the clock H’2 is (taking t’0 = t0) :
The gap t’2-t2 is negative thus the clock H’2, which has been set thanks to the formula , in reality is slow.
For N clocks regularly spaced by the same distance L' in R' that we believe to be synchronized in R’ by the method proposed by Clifford Will, we actually get N unsynchronized clocks in the Preferred Frame of Reference.
What is apparently synchronized in the referential of the train (the clocks) is actually not synchronized in the Preferred Frame of Reference and what is apparently not synchronized in the referential of the train (the arrival time of the signals emitted by the source) is actually synchronized in the Preferred Frame of Reference which is the REFERENCE.
Critics of what is developed in this paragraph will argue that Einstein's theory is correct since applied to the GPS that requires great precision and it works very well.
Indeed, the GPS system works very well because Einstein’s theory of special relativity is consistent.
The systematic errors compared to the Preferred Frame of Reference are retained and all the calculations remain consistent.
Even if one mistakenly thinks that two clocks are synchronized in a referential, if the first clock emits an electromagnetic signal at a time known by the two clocks, the time of receipt of the signal by the second clock will allow to deduce the distance separating the two clocks.
Finally, special relativity and the theory of the Preferred Frame of Reference make the same predictions, give the same results of experiments mainly due to the transitivity of the Lorentz transformation (we will return to this point deeper in the two chapters concerning the equivalence of the Lorentz transformation and the Doppler effect for the light).
3.11 Impossibility to obtain spatially separated clocks synchronized by a slow moving
Some people will argue that other methods can synchronize two distant clocks without using electromagnetic waves.
An often proposed idea is to bring together in one spatial point several clocks, synchronize them and then slowly move the clocks to their final position.
It is immediate to see that this method can not work. Indeed, as soon as one clock is moved with respect to another, its period becomes different from the other. If we have to separate the two clocks by a distance D, whether one of the two clocks is moved quickly for a short time or whether the clock is moved very slowly over a long duration, the final result will be the same: the clocks will be de-synchronized.
We can better explain by considering two clocks H1 and H2 located initially in the same inertial reference frame R1 with the speed V1 relative to the Preferred Frame of Reference. Then we give to the clock H2 the speed with regard to the Preferred Frame of Reference.
When the clock H2 will have reached its final position, the time difference between the two clocks, seen by an observer in the Preferred Frame of Reference will be the following, knowing that in the Preferred Frame of Reference the experiment will have lasted :
Taking account that, in the Preferred Frame of Reference, the classical composition of the speeds is valid, we can write : where refers to the speed of the clock H2 with regard to the clock H1 expressed in the Preferred Frame of Reference.
Supposing that the clock H2 is moving very slowly with regard to the clock H1 (that is to say V2/1 << V1), we can write at the first order :
Still at the first order, we can write :
that is to say :
Hence the final result : .
We find back the famous term obtained during the method of synchronization of clocks using light signals.
Note again that, the obtained difference in time depends on the sign of the speed V2/1 that is to say of the sense of moving of the clock H2 with regard to the clock H1.
Depending on whether the clock H2 is moving « toward the left » or « toward the right » with regard to the clock H1, the differences in duration will be opposite to one another.
This remark is fundamental:
- In the framework of special relativity, if we consider a clock H in an inertial reference frame R, then the emission of a light signal toward a clock situated on the left is equivalent to the transmission of a light signal toward a clock situated on the right. Similarly, moving a clock Hg to the left is equivalent to moving a clock Hd to the right;
- In the frame of the theory of the Preferred Frame of Reference, it is quite different. For a clock in the inertial reference R, it is not equivalent to emit a light signal to the left or to the right. Similarly, it is not equivalent to move a clock to the left or to the right. The reason is that the referential R itself is moving relative to the Preferred Frame of Reference that is deducted from the medium for the propagation of light.
3.12 Physical interpretation of the Lorentz transformation
The purpose of this section is to provide a demonstration / interpretation of the Lorentz transformation as physical as possible unlike many demonstrations based on hyperbolic cosinus and sinus which I find too mathematical and not physical enough.
This demonstration will be conducted in four phases:
-
- Time dilation
-
- Length contraction
-
- Lorentz equation for the spatial coordinate x
-
- Lorentz equation for the time coordinate t.
3.12.1 Time dilation
To establish this time dilation, we will use what is called a light clock.
A light clock is an apparatus provided with a light source (a laser, for example) that emits a light beam perpendicular to its motion and a mirror positioned perpendicular to the light beam at a distance D from the source.
This device can be compared to a clock whose period corresponds to a go and return of the light beam.
We assume the existence of two clocks H1 and H2 fixed in the Preferred Frame of Reference PFR and a clock H’ fixed in the referential R' moving at the speed V relative to PFR.
When we reason in the Preferred Frame of Reference, we handle the "real", physical periods of the clocks. The periods of the clocks H1, H2 and H 'determined in the Preferred Frame of Reference are "real". Thus, we will call them respectively T1true, T2true and T'true.
In the Preferred Frame of Reference, the period of the clock H 'satisfies the following equation:
whereas the period of the clocks H1 et H2 satisfies .
We can deduce the relation between the two “true” periods : where .
The period of the clock H' is "true" because it corresponds to the path of the light beam that is physically traveled in the Preferred Frame of Reference.
Note: for Einstein the speed of the light beam is equally c in the reference frame R'. This leads to a period of the clock H' in R' which is only apparent and equal to : .
For a full development of this argument see paragraph 6.5 Luminuous clock (with LASER).
Important remark :
We must assimilate that there is the instrument (a clock) and the measurement:
- The instrument (clock) has a period depending on its speed relative to the Preferred Frame of Reference as :
- The measurement of the time Dt with the clock in the PFR is related to the measurement of time Dt' with the clock in R' by
- For in the end the absolute time is the same for all Galilean frames : .
3.12.2 Length contraction
The same remark done about the time applies to lengths that is to say that we have :
where Ltrue is the length in the PFR of a fixed rule in the PFR, L’true is the length in the PFR of a rule of the same construction fixed in R', Dl is the measurement made in the PFR with the rule fixed in the PFR and Dl’ is the measurement made in the PFR with the rule fixed in R'.
Assume the existence of a stick B fixed in the Preferred Frame of Reference PFR and a stick B’ fixed in the inertial frame R'. The referential R' is moving at the speed V relative to the Preferred Frame of Reference.
It is assumed that at time t0 = t'0 = 0 the left end of the stick B and the right end of the stick B' are at the same abscissa x0 = 0.
Thus, the experiment consists in the cross of two sticks of the same construction in the space, their motion being parallel to the abscissa axis.
It is assumed that at time tf in the Preferred Frame of Reference t'f in R’ the left end of the stick B' is in x0 = 0.
Seen by an observer in the Preferred Frame of Reference:
the stick B 'moved for a duration : where DlB’/PFR is the measurement of the stick B’ in the Preferred Frame of Reference.
Seen by an observer in the referential R' :
the point of abscissa x0 in the PFR has moved of the measurement of the stick B’ in R' for a duration : .
To understand this last equality it is important to realize that the stick and the rule being in R’ are contracted by the same factor relative to the stick and the rule in the Preferred Frame of Reference, so the measurement of the stick B' in R' with a rule in R’ gives the same result as the measurement of the stick B in the PFR with a rule in the PFR.
This measure is: .
Similarly one could write : .
From the two equations giving Dt and Dt’ we deduce the following relation : .
Yet the relation allows us to obtain the following formula : .
Finally, knowing the relation (completely written in the Preferred Frame of Reference) between the instrument (the rule) and the measure, we obtain the following formula : which means that the rule fixed in R’ but moving at the speed V with regard to the Preferred Frame of Reference is affected by a real physical contraction of a factor g (still with regard to the Preferred Frame of Reference which is the REFERENCE).
Remark : it would seem more natural to write the relation which would give a opposite final result ( and ) but it is well the two following crossed relations which are valid :
3.12.3 Lorentz equation concerning the spatial coordinate x
To establish the relationship on the distances, we introduce three referentials :
- The Preferred Frame of Reference PFR;
- Any inertial frame R' moving at the speed V = VR’/PFR relative to the PFR;
- A referencial R’* bound to the referential R’ but owning rules of the same length as in the PFR and clocks of the same period as those in the PFR.
For an event (xPFR , tPFR) in the Preferred Frame of Reference, we have in the referential R’* :
(the classic composition of the speeds can be simply applied).
As the rules in R’ are shorter by a factor with regard to the rules in R’* we have : .
3.12.4 Lorentz equation concerning the temporal coordinate t
In order to establish the relation on the durations, we simply go back to the case of the paragraph 3.10 concerning the synchronization of two clocks in R’.
Considering that the clocks in R’ have a period larger than the ones in PFR, we have seen that we obtain the following relation in the Preferred Frame of Reference PFR :
. with whence we obtain .
Finally, the Lorentz transformation can be written :
.
From these two relations, we can easily deduce :
-
xPFR in order to obtain the couple (xPFR, tPFR) ;
-
t’ in order to obtain the couple (x’, t’).
For example, from the relation we can deduce .
By replacing the time tPFR by its expression, we obtain : .
So we have .
Yet whence .
Thus, the Lorentz transformation can be written this way : .
3.13 Transit time of the light in order to obtain the absolute chronological order of the events
It has been seen previously that it was impossible to really synchronize several distant clocks even if they all belong to the same inertial referential.
Thus it is impossible to use several observers/clocks belonging to the same inertial referential to determine the chronological order or the simultaneity of events.
This fact imposes to resort to only one observer to determine the chronological order of events. This unicity of the observer is one of the key points of this paragraph.
Moreover, this paragraph tries to show why the chronological order of the events is relative to the referential of the observer in Einstein’s special relativity and absolute in the theory of the Preferred Frame of Reference.
Consequently, I am going to introduce what I call « the light clock » which is constituted of a LASER and a mirror. Whenever the ray of light carries out a round trip between the LASER and the mirror, one cycle or one period is counted.
A very interesting case is the one when the direction of the light path is perpendicular to the speed vector of the moving clock.
For example, supposing that the light clock is in a train, the LASER will be put down on the ground, the mirror will be stuck on the ceiling of the train and the path of the ray of light will carry out vertically from the ground towards the ceiling, then from the ceiling towards the ground after reflection on the mirror.
Let’s suppose that the railway owns a light clock and that the train owns its light clock.too.
The two following figures sum up Einstein’s vision on these two light clocks :
These two figures show the perfect symmetry of Einstein’s vision between the observer on the ground and the observer in the train :
-
the observer on the ground will see the round trip of the ray of light of its clock vertical and will declare that he measures the period of the clock on the train as bigger. The observer on the ground will declare that the return of the ray of light of its clock has occurred BEFORE the return of the ray of light of the clock in the train;
-
the observer in the train will see the round trip of the ray of light of its clock vertical and will declare that he measures the period of the clock on the ground as bigger. The observer in the train will declare that the return of the ray of light of its clock has occurred BEFORE the return of the ray of light of the clock on the ground.
For the same events (return of the ray of light of the clock on the ground and return of the ray of light of the clock in the train), the observer on the ground and the observer in the train will declare a reverse chronological order.
Now, let’s examine the same experiment in the framework of the theory of the Preferred Frame of Reference for which the reality of an experiment has always to be determined in the Preferred Frame of Reference. Thus, when we want to know what really happens for an observer in any referential, we have to analyse the events in the Preferred Frame of Reference.
Let’s suppose that the ground (the railway) be linked to the Preferred Frame of Reference and that a referential be linked to the referential of the train.
In the Preferred Frame of Reference, we will have the following sight :
I would like to show that the observer in the train believes that the return of the ray of light of his clock occurs before the return of the ray of light produced by the clock on the ground because it doesn’t take into account the distance between the two points of return D = OgroundOtrain traveled by the ray of light of the clock of the ground after its round trip in order to reach the observer of the train.
Einstein’s method for establishing the simultaneity of events or their chronological order being based on light signals, we must :
-
make the reasoning for (chosen on the ground or in the train) and who stands (spatially or temporally) at the beginning or at the end of a cycle of its own clock ;
-
take into accountthe path travelled by the light so that the information on the return of the ray of light of the other clock reaches him.
In the Preferred Frame of Reference :
-
the period of the clock on the ground is : ;
-
the period of the clock of the train is : withV = Vtrain/ground.
1st case : the observer is standing at the departure of the ray of light of the clock of the train, then at the arriving of the ray of light of the clock of the train and goes on its movement united with the train (point Otrain).
We call TX the duration that is needed for the ray of light of the clock of the ground to travel a round trip, then reach the point Otrain (red path in dotted line).
This duration TX has to be seen as the time taken by the light to travel one round trip of the clock of the ground (corresponding to one cycle) then to inform the point Otrain that the cycle is finished.
We have which means that TX > Ttrain and that the duration for the light to travel a round trip of the clock of the ground, then to “inform” the point Otrain that the cycle of the clock of the ground is finished, is superior to the cycle Ttrain of the clock of the train even thought .
This reasoning shows why the observer of the train has the feeling that the round trip of the ray of the clock of the ground finishes AFTER the end of the round trip of the clock of the train (Tx > Ttrain even though, actually, in the Preferred Frame of Reference, Ttrain > Tground).
2nd case : the observer is standing at the outset of the ray of light of the clock of the train then at the arrival of the ray of light of the clock of the train then stops in the Preferred Frame of Reference in order to wait for the information coming from the clock on the ground.
We still call TX the duration that is needed for the ray of light of the clock of the ground to make a round trip, then to reach the point Otrain (red path in dotted line).
In this case, we have : .
Let’s calculate the time difference between :
We have which means that TX > Ttrain and that the duration for the light to travel a round trip of the clock of the ground, then to “inform” the point Otrain that the cycle of the clock of the ground is finished, is superior than the cycle Ttrain of the clock of the train even thought .
Remark : in the following figure, all the paths are travelled at the speed of light, so the inequality which has been demonstrated is equivalent to a purely geometrical inequality :
distance red path in dotted line > distance green path in solid line (I)
This last inequality being true means that the red path in dotted line is always greater than the path in green in solid line, that is to say that we have : (the observer of the train sees the return of the ray of light of the clock of the ground after the return of the ray of light of the clock in the train) even though (since ).
3.14 Apparent effects versus real physical effects
One of the main differences between Einstein’s vision and Lorentz’s vision concerning the contraction of the lengths and the dilation of the durations is as follows :
-
for Einstein the effects are apparent, a little bit in a similar way as an optical effect ;
-
for Lorentz (and other physicists as Fitzgerald, Larmor, Voigt), the effects are physical and real.
It is possible to consider that the Special Relativity is not limited to uniform rectilinear movements but to all the possible movements (accelerations, turns, …).
Indeed, it is possible to decompose any kind of movement in several short elementary sections, each of whom is equivalent to a uniform rectilinear movement for which the Lorentz transformation is appropriate.
The global effect combined to the whole movement will be the “sum” of all the effects obtained with the application of the Lorentz transform. If the effects are apparent for each section of the trajectory, so it is legitimate to think that they will be also apparent for the whole trajectory.
This reasoning makes the real physical gap between two atomic clocks subjected to different speeds surprising, in the frame of a theory where the effects are apparent.
We can also quote the experiment of disintegration of muons. Besides with regard to a terrestrial referential (a laboratory), they have a rather short lifetime (about 2.2 µs) but this lifetime hugely increases when the muons arrive with a very high speed from space towards the surface of the Earth. It is this phenomenon which allows them to reach the surface of the Earth. If this temporal dilation wasn’t real, physical, the muons wouldn’t really, physically touch the surface of the Earth.
3.15 Conclusion
This paragraph tries to draw some teaching from everything seen in this chapter.
The first teaching that can be held back is that Einstein’s method to determine the simultaneity of two events is comparable to a theory of transmission of signals.
I mean that it is a question of emission and reception of signals and about the moments when we transmit and receive these signals.
Thus, what is important is the spatial position of the source at the moment of the emission and then, the spatial position of the source is no longer important at all.
Likewise, what is only important is the spatial position of the receiver at the moment of the reception, before this moment, the spatial position of the receiver has no importance.
Moreover, Einstein tries to convince us that it is because an observer standing at the point M’ on the train moves with regard to the two flashes of lightening which have occurred at the two points A and B of the railway that he doesn’t see the two flashes simultaneously. But, at better thought, it is not the movement that implies a different arrival of the two signals but the position of the observer at the moment of the arrival of the two signals.
Thus, if we consider two simultaneous events (two flashes of lightening) which occur at the points A and B of a given referential, these two points define three zones :
-
a mediator plan of the points A and B determining a set of spatial points for which an observer will see the two flashes of lightening simultaneously ;
-
a “semi-space” delimited by the mediator plan and containing the point A which determines a set of spatial points for which some observers will see the flash of lightening coming from A before the flash of lightening coming from B ;
-
a “semi-space” delimited by the mediator plan and containing the point B which determines a set of spatial points for which some observers will see the flash of lightening coming from B before the flash of lightening coming from A ;
The second teaching concerns my method which is liable to inform about the chronological order of the events to any observers.
The fundamental base of this method is to be able to dispose of two synchronized clocks belonging to the Preferred Frame of Reference.
If we accept that it is possible to synchronize two distant clocks belonging to the Preferred Frame of Reference, then the absolute nature of the simultaneity seems obvious.
Indeed, these two synchronized clocks can generate as many pairs of events as wanted which will be simultaneous (for example, at any time, the two clocks activate a flash). It is known beforehand that the events will be simultaneous because they are generated by synchronized clocks.
Moreover, associated to each pair of simultaneous events, the two clocks can also transmit an electromagnetic signal containing the information of the time of emission.
Any observer who will receive the two signals sent by the two clocks, will have the choice :
-
he can only take into account the moment of the arrival of the signals. As the king in my story, he only takes into account the moment of the arrival of the messengers ;
-
he can be interested by the information contained by the electromagnetic wave, that is to say, as the king’s counsellor, listen to the message delivered by the messenger.
Note : the reader can wonder why I systematically speak about Galilean referentials. The reason is that they are referentials for which the clocks keep the same period. Keeping a constant distance between two clocks is not a sufficient criteria. The best proof consists in putting a clock in the center of a rotating disk and the second clock on a point of the periphery.
The third and most important teaching of this chapter is the following one :
-
the true and physical simultaneity between two events is ABSOLUTE and doesn’t depend on the observer ;
-
the method given by Einstein which leads to a simultaneity relative to the observer is in fact an apparent “non-simultaneity” due to the finite speed of light.